Renewable Energy Advancements Across the Globe
Advanced control methods are being developed to harness wind energy at high altitudes. These methods involve controlling tethered airfoils to optimize energy generation, which could lead to a significant increase in renewable energy output
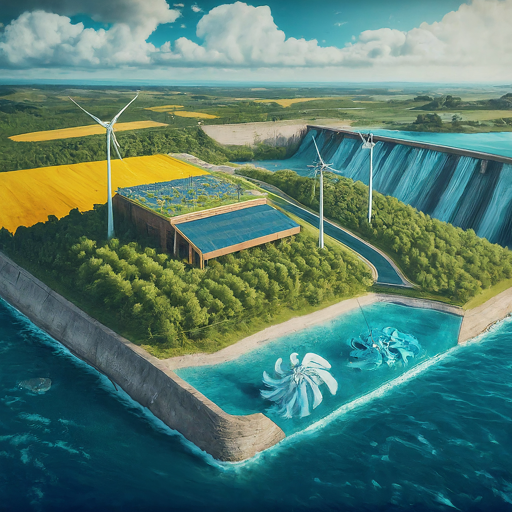
1. High-Altitude Wind Energy Generation
Advanced control methods are being developed to harness wind energy at high altitudes. These methods involve controlling tethered airfoils to optimize energy generation, which could lead to a significant increase in renewable energy output[5].
Pros:
- High-altitude winds are generally stronger and more consistent compared to ground-level winds, providing a more reliable source of renewable energy[5].
- Tethered airfoils can access wind resources at higher altitudes without the need for large, expensive tower structures required for traditional wind turbines[5].
- The advanced control methods allow for precise positioning and orientation of the tethered airfoils to maximize energy capture, improving the overall efficiency of the system[5].
Cons:
- The technology is still in the research and development stage, and large-scale commercial deployment may take several years to achieve[5].
- Maintaining the stability and control of the tethered airfoils in high-altitude conditions can be technically challenging and may require complex control algorithms[5].
- The infrastructure required for high-altitude wind energy generation, such as tethers, anchoring systems, and power transmission, can be costly and may face regulatory hurdles in some regions[5].
2. Green Credit and Carbon Neutrality
The role of green credit in promoting carbon neutrality through renewable energy firms has been studied. This research demonstrates the importance of financial incentives in driving technological innovation and the transition to a low-carbon economy[3].
Pros:
- Green credit policies can provide financial support and incentives for renewable energy companies to invest in research and development, leading to technological breakthroughs[3].
- Increased access to green credit can help renewable energy firms scale up their operations and compete more effectively with traditional fossil fuel-based energy providers[3].
- Green credit can contribute to the overall goal of carbon neutrality by encouraging the adoption of renewable energy sources and reducing greenhouse gas emissions[3].
Cons:
- The effectiveness of green credit policies in driving technological innovation and carbon neutrality may vary depending on the specific policy design and implementation[3].
- There is a risk of "greenwashing," where companies may exploit green credit policies without making meaningful progress towards sustainability[3].
- The allocation of green credit may be subject to political and economic factors, potentially leading to uneven distribution and missed opportunities for technological breakthroughs[3].
3. Solar Cell-Integrated Energy Storage Devices
Integrating solar cells with energy storage devices is a breakthrough for electric vehicles. This technology allows for efficient energy storage and utilization, enhancing the performance of renewable energy systems[4].
Pros:
- Combining solar cells and energy storage devices, such as batteries, can improve the overall efficiency and performance of electric vehicles by providing a more integrated and self-sufficient energy system[4].
- This technology can help reduce the reliance on the grid and increase the use of renewable energy sources, contributing to the decarbonization of the transportation sector[4].
- Integrated solar cell-energy storage systems can provide a more reliable and resilient power source for electric vehicles, especially in areas with limited grid infrastructure[4].
Cons:
- The integration of solar cells and energy storage devices can be technically complex, requiring advanced materials, control systems, and thermal management solutions[4].
- The cost of these integrated systems may be higher compared to traditional electric vehicle power systems, which could hinder widespread adoption in the short term[4].
- The scalability and mass production of these integrated systems may pose challenges, as the manufacturing processes need to be optimized for cost-effectiveness and reliability[4].
4. European Green Deal and Renewable Energy Transformation
The European Green Deal policy has been instrumental in promoting renewable energy generation in the European Union. This policy has led to a significant increase in the share of renewable energy in electricity generation, surpassing that of fossil fuels[4].
Pros:
- The European Green Deal has set ambitious targets for renewable energy deployment, driving investment and technological innovation in the sector[4].
- The policy has fostered a regulatory environment that supports the integration of large-scale renewable energy sources into the grid, enabling a more sustainable energy system[4].
- The increased share of renewable energy in the EU's electricity generation has contributed to a reduction in greenhouse gas emissions, aligning with the region's climate change mitigation goals[4].
Cons:
- The implementation of the European Green Deal has faced some challenges, such as the need for significant infrastructure investments, grid modernization, and the coordination of policies across member states[4].
- The transition to renewable energy may have uneven impacts on different sectors and regions, requiring careful management to ensure a just and equitable transformation[4].
- The long-term sustainability of the European Green Deal's renewable energy policies may depend on continued political support and the ability to adapt to changing market conditions and technological advancements[4].
5. Microbial Fuel Cells
Microbial fuel cells (MFCs) are a promising technology for renewable energy generation, particularly in wastewater treatment. MFCs utilize bacteria to transform organic matter into hydrogen gas or electricity, offering a sustainable and decentralized energy solution[5].
Pros:
- MFCs can generate renewable energy while simultaneously treating wastewater, providing a dual-purpose solution that can contribute to the circular economy[5].
- The technology is relatively simple and can be deployed in a decentralized manner, making it suitable for remote or off-grid applications[5].
- MFCs can operate using a wide range of organic feedstocks, including agricultural and industrial waste, making them a versatile renewable energy solution[5].
Cons:
- The power output of MFCs is generally low compared to traditional energy generation technologies, limiting their large-scale deployment for grid-scale electricity production[5].
- The scaling up of MFC systems can be challenging, as the performance and efficiency of the technology may be affected by factors such as reactor design, microbial community composition, and operating conditions[5].
- The long-term stability and durability of MFC systems need further research and development to ensure reliable and consistent energy generation[5].
6. Layered Double Hydroxides and Hydrogen Production
The synthesis of layered double hydroxides (LDHs) has led to a breakthrough in hydrogen production from clean/renewable sources. LDHs play a crucial role in overcoming the limitations of traditional hydrogen production methods, enabling high hydrogen evolution rates.
Pros:
- LDHs can be used as efficient catalysts or catalyst supports for water splitting, a process that generates hydrogen gas from water using renewable energy sources.
- The unique layered structure and tunable composition of LDHs allow for the optimization of their catalytic properties, leading to improved hydrogen production efficiency.
- The use of LDHs in hydrogen production can contribute to the development of a sustainable hydrogen economy, reducing the reliance on fossil fuel-based hydrogen generation.
Cons:
- The large-scale synthesis and deployment of LDH-based hydrogen production systems may require further technological advancements and cost reductions to be economically viable.
- The integration of LDH-based hydrogen production with other renewable energy technologies, such as solar or wind power, may pose technical challenges in terms of system design and optimization.
- The long-term stability and durability of LDH-based catalysts under operational conditions need to be thoroughly investigated to ensure the reliability of the hydrogen production process.
7. Optimal Sizing of Hybrid Renewable Energy Systems
The optimal sizing of hybrid renewable energy systems, considering power sharing and electric vehicles, is a significant advancement in renewable energy system design. This approach improves system performance and reduces costs.
Pros:
- Optimal sizing of hybrid renewable energy systems, which combine multiple renewable energy sources (e.g., solar, wind, and energy storage), can lead to more efficient and cost-effective energy generation.
- Incorporating power sharing and electric vehicle integration into the system design can enhance the overall flexibility and responsiveness of the renewable energy system, improving its ability to meet dynamic energy demands.
- The optimization of hybrid renewable energy systems can help reduce the reliance on fossil fuels and contribute to the decarbonization of the energy sector.
Cons:
- The optimal sizing and design of hybrid renewable energy systems can be a complex and computationally intensive process, requiring advanced modeling and optimization techniques.
- The integration of power sharing and electric vehicles into the system design may introduce additional technical and operational challenges, such as grid stability, load management, and communication protocols.
- The deployment of optimized hybrid renewable energy systems may require significant upfront investments, which could hinder their widespread adoption, especially in regions with limited financial resources.
Citations:
[1] https://arxiv.org/abs/2401.17814
[2] https://www.semanticscholar.org/paper/0513ac411a6f8319b8d2a7509e934ffccd9130ad
[3] https://www.semanticscholar.org/paper/6e28b53d659de3ba110e3ebd73521858fa872e5d
[4] https://www.semanticscholar.org/paper/80dba2a2cd21731a4fecdafb0b425f924b26746e
[5] https://www.semanticscholar.org/paper/a4041db3a29bed84bdf446e808d2d11bce6c3be4